Preserving Native Components of an Amniotic Suspension Allograft:
In Vitro Evidence of Anti-Inflammatory and Bioactive Cellular Responses
In Vitro Evidence of Anti-Inflammatory and Bioactive Cellular Responses
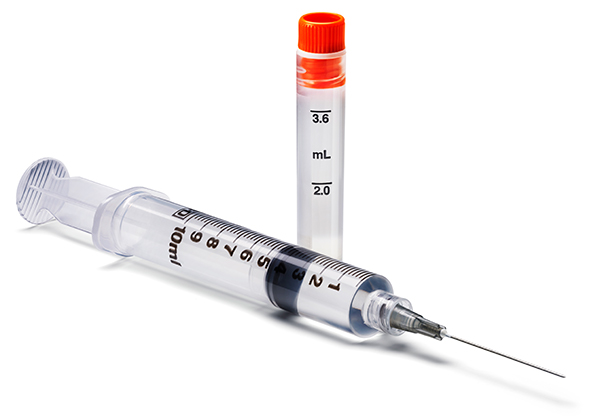
Injectable amnions or amniotic suspension allografts (ASA) are a popular topic of conversation in the foot and ankle arena. Foot and ankle injuries can be debilitating and render a patient “mobility impaired”. Once degenerative changes take place, tendinosis and/or osteoarthritis can elicit pain and discomfort leading to patients seeking assistance for a solution-based remedy to improve function, mobility, and decrease pain. Achilles tendinopathy, plantar fasciitis, posterior tibial tendinosis, peroneal tendinosis, ankle arthritis, first MPJ arthritis, as well as subtalar joint arthritis (just to name a few) can cause significant impaired mobility and pain. Injectable amnions have been utilized for some of the above stated conditions, and it is believed that preserving the function of the native amniotic tissue is important in order to have a clinical benefit. This article describes generated evidence which demonstrates how preserved bioactive native components of an amniotic suspension influence the decrease of inflammation and cellular responses in vitro.
MSCs, growth factors, and cytokines are found in fetal annexes such as cord blood, placenta, amniotic membranes, and amniotic fluid (1). Low immunogenicity, antifibrotic, anti-inflammatory, and angiogenic properties make placental tissues an attractive option for various procedures and tissue engineering, while avoiding ethical concerns of embryonal tissue, as placental tissue will be discarded and disposed, if not utilized for allografting (2,3). Human amniotic suspension allografts (ASA, NuCel®, Organogenesis Inc. and ReNu®, Organogenesis, Canton, MA) are cryopreserved suspensions, which contain micronized amniotic membrane, amniotic fluid cells, and are processed according to the Food & Drug Administration (FDA) Good Tissue Practices (GTPs) and American Association of Tissue Banks (AATB) standards (4,5). All donated ASA placental tissue is tested for HIV, HEP B and C, Human T-lymphotrophic virus I&II, and syphilis (4,5).
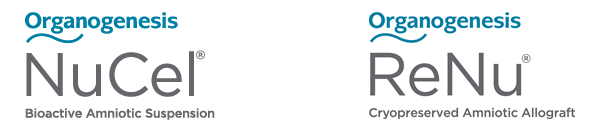
In vitro preclinical data allows us to monitor the bioactive cellular responses of particular cell types when exposed to ASA, demonstrating the preservation of biological activity of the tissue product. MSC migration and MSC proliferation has been shown to significantly increase when exposed to dose dependent ASA in vitro (4). MSCs have been isolated from almost all adult tissues and release growth factors (GF) and cytokines with immunomodulatory effects after injury, which provides a microenvironment with specific molecules through paracrine signaling (6,3,7). These signals affect a variety of physiological processes such as cell proliferation, differentiation, and angiogenesis (8).
Tenocyte density has also shown to increase significantly when tenocytes are exposed to ASA in vitroat various concentrations, and tenocyte migration significantly increases when exposed to ASA at 50% concentration in vitro(9).Increased tenocyte migration and density is beneficial throughout the phases of tendon healing as tendons are not physiologically conducive to healing due to limited vascularity (10). Tenocytes naturally exist in low populations, have a low metabolic rate, and consume 1/7th the oxygen as muscle cells, which also contributes to the difficulty of tendon healing (10). Increasing the density of tenocytes improves the efficiency of healing to repair the cellular scaffold, which tendons require (11).
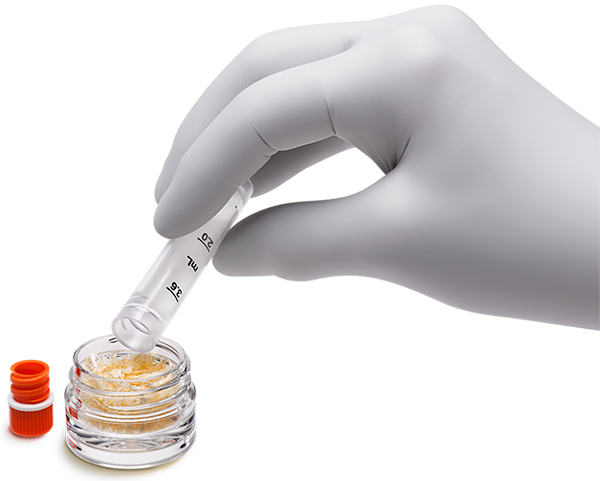
Tenocyte extracellular matrix (ECM) deposition has also been monitored in vitro. When tenocytes are exposed to 50% ASA cultured media in vitro, an increase of ECM deposition of collagen, elastin, and sGAGs have all been seen at day 7 and 14 (9). Tenocyte ECM deposition is a significant process during tendon healing, as alterations in ECM, such as glycation, inflammation, and other patient comorbidities can decrease the mechanical integrity of the tendon (12).
Tenocyte response to inflammatory cytokine stimulation (TNF-α and IL-1β)is also positively altered when exposed to ASA in vitro, resulting in reduced gene expression of IL-8 and TGF-1β (9). Reducing IL-8 decreases neutrophil activation; therefore, altering potential damaging effects within the soft tissue, while decreasing TGF-1β is known to decrease scarring and adhesions in tendon and soft tissue (13,14). Decreasing damaging effects within the tendon, decreasing scarring, and adhesions is beneficial as often healing tendons fail to adapt to recurrent excessive loading since scar tissue is weaker than original tendon tissue (10).
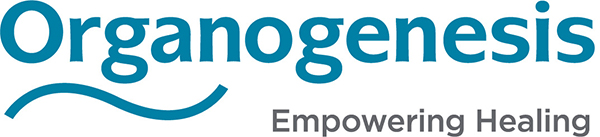
-
Lanfranchi A, Porta F, Chirico G. Stem cells and the frontiers of neonatology. Early Hum Dev. 2009 Oct 85(10 suppl):S15-s18.
Follow This Link -
Niknejad H, Peirovi H, Jorjani M, et al. Properties of the amniotic membrane for potential use in tissue engineering. Eur Cell Mater. 2008 Apr 29;15:88-99.
Follow This Link -
Friel NA, de Girolamo L, Gomoll AH, et al. Amniotic Fluid, Cells, and Membrane Application. Operative Tech in Sports Medicine. 2017 Mar;25(1):20-24.
Follow This Link -
Data on file. Document No: NuCel-504
-
Vines JB, Aliprantis AO, Gomoll AH, et al. Cryopreserved Amniotic Suspension for the Treatment of Knee Osteoarthritis. J Knee Surg. 2016 Aug;29(6):443-450.
Follow This Link -
De Girolamo L, Lucarelli E, Alessandri G, et al. Mesenchymal stem/stromal cells: A new cells as drugs paradigm. Efficacy and critical aspects in cell therapy. Curr Pharm Des. 2013;19(13): 2459-2473.
Follow This Link -
Murray IR, Corselli M, Petrigliano FA, et al. Recent insights into the identity of mesenchymal stem cells: Implications for orthopaedic applications. Bone Joint J. 2014 Mar;96-B(3):291-298.
Follow This Link -
Nicola N. Guidebook to cytokines and their receptors. Oxford; New York: Oxford University Press;1994.
Follow This Link -
Kimmerling KA, McQuilling JP, Staples MC, Mowry KC. Tenocyte cell density, migration, and extracelluar matrix deposition with amniotic suspension allograft. J Ortho Research 2019 Feb;37(2):412-420.
Follow This Link -
Sharma P, Maffulli N. Tendon Injury and Tendinopathy: healing and repair. J Bone Joint Surg Am. 2005 Jan;87(1):187-202.
Follow This Link -
Liu CF, Aschbacher-Smith L, Barthelery NJ, et al. 2011. What we should know before using tissue engineering techniques to repair injured tendons: a developmental biology perspective. Tissue Eng Part B Rev. 2011 Jun;17(3):165-176.
Follow This Link -
Ahmed AS. Does Diabetes Mellitus Affect Tendon Healing? Adv Exp Med Bio. 2016;920:179-184.
Follow This Link -
Ackermann PW, Domeij-Arverud E, Leclerc P, et al. 2013. Anti-inflammatory cytokine profile in early human tendon repair. Knee Surg, Sport Tr A. 2013 Aug;21(8):1801-1806.
Follow This Link -
Katzel EB, Wolenski M, Loiselle AE, et al. Impact of Smad3 loss of function on scarring and adhesion formation during tendon healing. J Orthop Res. 2011 May;29(5):684-693.
Follow This Link
Comments
There are 0 comments for this article